Lenalidomide and pomalidomide potently interfere with induction of myeloid-derived suppressor cells in multiple myeloma
Y.S. and J.K. contributed equally to this study.
Summary
An increase in immunosuppressive myeloid-derived suppressor cells (MDSCs) is associated with disease progression and treatment resistance in multiple myeloma (MM). We investigated the mechanisms underlying MDSC induction, and sought to discover a strategy for prevention of MDSC induction in MM. Using a transwell co-culture system, four of nine examined human myeloma-derived cell lines (HMCLs) were potent in inducing monocytic (M)-MDSCs from normal peripheral blood mononuclear cells (PBMCs). As the results, we identified that secretion of C-C motif chemokine ligand 5 (CCL5) and macrophage migration inhibitory factor (MIF) by myeloma cells is a prerequisite for induction of MDSCs in MM. The immunomodulatory drug (IMiD) compounds, such as lenalidomide (LEN) and pomalidomide (POM), were identified as potent inhibitors of MDSC induction through bidirectional molecular effects of cereblon (CRBN)-dependent and -independent downregulation of CCL5 and MIF in myeloma cells; and downregulation of C-C motif chemokine receptor 5, a receptor for CCL5, and induction of interferon regulatory factor 8, a critical transcription factor for monocytic differentiation, in PBMCs. In the present study of the molecular mechanisms underlying MDSC induction, we identified a novel effect of LEN and POM of inhibiting MDSC induction via overlapping regulatory effects in myeloma cells and normal PBMCs.
Myeloid-derived suppressor cells (MDSCs), which are largely classified into polymorphonuclear (PMN)-MDSCs and monocytic (M)-MDSCs, associate with disease progression, treatment failure, and poor prognosis in various cancers.1-4 In a tumour microenvironment, MDSCs are induced by co-localising tumour cells, and, in turn, impair tumour immune surveillance by activating immunosuppressive effector cells, such as regulatory T cells (Tregs), and suppressing cytotoxic T cells and natural killer (NK) cells. MDSCs also promote tumour cell proliferation,2, 3 while some anti-cancer drugs increase MDSC function by promoting a focal inflammation.3, 5
Multiple myeloma (MM) remains as a mostly incurable disease, despite the recent progress of immunochemotherapy using immunomodulatory drugs (IMiD compounds), such as lenalidomide (LEN) and pomalidomide (POM), and/or monoclonal antibodies (Abs), such as those against CD38 or signalling lymphocytic activation molecule family member 7 (SLAMF7).6-10 As with other cancers, MDSCs increase along with disease progression and with the acquisition of treatment resistance in MM.11-13 In the present study, we investigated the mechanism underlying MDSC induction from normal peripheral blood mononuclear cells (PBMCs), and tried to discover a moiety that could block MDSC induction in MM.
Patients and methods
Cell lines, reagents and patient-derived samples
Human myeloma-derived cell lines (HMCLs), AMO1, NCI-H929, OPM2 and LP-1 (Deutsche Sammlung von Mikroorgranismen und Zellkulturen GmbH, Braunschweig, Germany), RPMI8226 and IM9 (American Type Culture Collection, Manassas, VA, USA), and KMS-12-BM, KMS-28-PE and KMS-34 (Dr Ohtsuki, Kawasaki Medical School, Kawasaki, Japan), and regents utilised were described in Data S1. Generation of 15a, a cereblon (CRBN) homo-proteolysis targeting chimera (PROTAC) compound, and CRBN-6-5-5-VHL, a CRBN-VHL hetero-PROTAC compound, that combine CRBN with another E3 ligase and degrade CRBN has been described elsewhere.14, 15 PBMCs and serum samples were collected from patients with MM with written informed consent.
Induction of MDSCs from PBMCs by indirect co-culture with HMCLs and pharmacological intervention
Indirect co-culture of HMCLs and PBMCs was performed using a six-well transwell cell culture plate. Briefly, 1 × 105 HMCLs seeded in the upper Cell Culture Insert with 1·0 μm pores (Corning, NY, USA) and 1 × 106 PBMCs in the lower chambers were indirectly co-cultured for 5 days (Fig 1A). To search for the moiety that could prevent MDSC induction, we examined the effects of all-trans retinoic acid (ATRA), maraviroc (MVC), celecoxib and common anti-myeloma agents, such as LEN, POM and a proteasome inhibitor (PI) bortezomib (BTZ), on MDSC induction, referring to observations and controversies in previous basic and clinical researches.13, 16-20
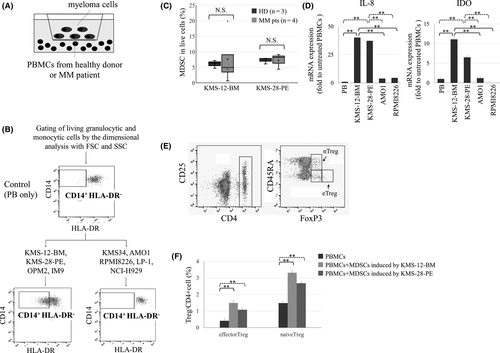
Flow cytometric analysis
CD14+/CD33+/HLA-DR- M-MDSCs and CD14−/CD15+/CD33+/HLA-DR- PMN-MDSCs derived from PBMCs were evaluated by flow cytometric analysis (FACS Canto II; BD Biosciences, San Jose, CA, USA). Naïve and effector regulatory Tregs were defined as CD45RA+Foxp3low CD4+ and CD45RA−Foxp3high CD4+ cells, respectively (Data S1).
Cytokine assays
A Proteome Profiler Human Cytokine Array Kit (ARY005B) (R&D Systems, Minneapolis, MN, USA) was used for detection of 36 soluble factors in conditioned medium (CM) of HMCLs (Fig S1). The concentrations of interleukin 8 (IL-8), indoleamine 2, 3-dioxygenase (IDO), macrophage inflammatory protein-1α (MIP-1α), chemokine (C-C motif) ligand 5 (CCL5) and macrophage migration inhibitory factor (MIF) were measured using enzyme-linked immunosorbent assay (ELISA) kits (R&D Systems).
Quantitative reverse transcription-polymerase chain reaction (qRT-PCR)
Methods for total RNA isolation and qRT-PCR, and the primers utilised are described in Data S1.
Western blotting
Western blotting was performed21 using primary Abs against CRBN (HPA045910) (Sigma-Aldrich, St. Louis, MO, USA), Ikaros family zinc finger protein 1 (IKZF1; A303-516A) (Bethyl Laboratories, Montgomery, TX, USA), IKZF3 (15103) (Cell Signaling, Danvers, MA, USA) and β-actin (Sigma-Aldrich).
Statistics
Methods for statistical analyses are described in Data S1.22 A P < 0·05 was considered statistically significant.
Results
Specific HMCLs induce M-MDSCs from normal PBMCs
We first investigated if the presence of HMCLs caused induction of MDSC from normal PBMCs from three healthy donors (HDs) using both direct and indirect co-culture systems. MDSCs were undetectable in normal unstimulated PBMCs, but four (KMS-12-BM, KMS-28-PE, OPM2 and IM9) of the nine HMCLs examined were able to induce M-MDSCs, but not PMN-MDSCs (Fig 1B,C). The indirect co-culture was more potent in inducing M-MDSCs compared with the direct co-culture (data not shown), perhaps because the survival/proliferation of normal PBMCs was competitively disturbed by the overgrowth of HMCLs when directly co-cultured for 5 days. Therefore, we used indirect co-culture as the optimal model throughout this study. M-MDSCs were also inducible from PBMCs from four patients with MM by the co-culture with KMS-12-BM or KMS-28-PE cells, and the degrees of M-MDSC induction from PBMCs of patients with MM were not significantly different from those from HDs (Fig 1C; Fig S2).
To confirm the functional relevance of induced M-MDSCs, we isolated the CD33-positive myeloid fraction of which approximately 50% cells were M-MDSCs induced from PBMCs after co-culture with KMS-12-BM or KMS-28-PE cells (data not shown), and examined its molecular and biological features by comparing those of CD33-positive myeloid fraction with no detectable MDSCs after co-culture with MDSC-non-inducible HMCLs as the negative control. Cells of MDSC-containing CD33-positive fractions after co-culture with MDSC-inducible HMCLs (KMS-12-BM and KMS-28-PE) cells showed significant transcriptional upregulation in levels of IDO and IL-8 than unstimulated PBMCs and cells of CD33-positive fractions after co-culture with MDSC-non-inducible HMCLs (AMO1 and RPMI8226) (Fig 1D). In addition, co-culture with cells of MDSC-containing CD33-positive fractions induced more Tregs in PBMCs compared with untreated PBMCs (Fig 1E,F), while this was not the case with MDSC-absent CD33-positive fractions after co-culture with MDSC-non-inducible HMCLs (data not shown). These indicate that MDSCs induced by co-culture with specific HMCLs possess immunosuppressive properties. Unexpectedly, however, the concentrations of IDO and IL-8 in CM after the co-culture with MDSC-inducible HMCLs and those after the co-culture with MDSC-non-inducible HMCLs were not significantly different (Fig S3), suggesting the co-presence of IDO and IL-8 secretion independent of transcriptional upregulation in CD33-positive fraction.
Mechanism of MDSC induction by co-culture with HMCLs
Given that MDSCs were induced under the indirect co-culture system, we hypothesised that a soluble factor specifically and commonly secreted by the four MDSC-inducible HMCLs is critical for inducing MDSCs. By investigating CM from HMCLs using a cytokine array, CCL5 and MIP-1α were found to be secreted only by MDSC-inducible HMCLs, while MIF was secreted by all HMCLs examined (Fig 2A,B, Table 1). The concentration of CCL5 was significantly higher in CM from MDSC-inducible HMCLs than MDSC-non-inducible HMCLs. By contrast, MIP-1α (P = 0·27) and MIF (P = 0·4) levels in CM were not significantly different between MDSC-inducible and MDSC-non-inducible HMCLs (Fig 2C).
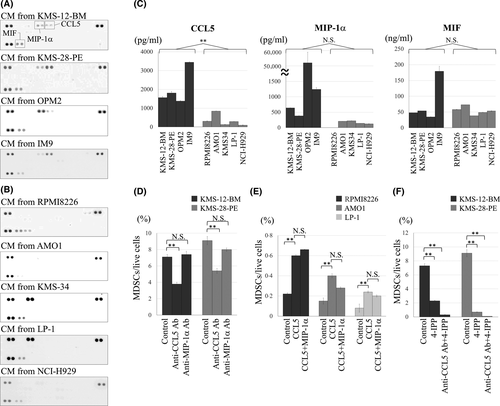
Soluble factor | MDSC-inducible HMCLs | Non-MDSC-inducible HMCLS | |||||||
---|---|---|---|---|---|---|---|---|---|
KMS-12-BM | KMS-28-PE | OPM-2 | IM9 | RPMI8226 | AMO1 | KMS34 | LP-1 | NCI-H929 | |
C5a | − | − | − | − | − | − | − | − | − |
CD40L | − | − | − | − | − | − | − | − | − |
G-CSF | − | − | − | − | − | − | − | − | − |
GM-CSF | − | − | − | − | − | − | − | − | − |
CXCL1 | − | − | − | − | − | − | − | − | − |
CCL1 | − | − | − | − | − | − | − | − | − |
ICAM-1 | − | − | − | − | − | + | − | − | − |
IFN-γ | − | − | − | − | − | − | − | − | − |
IL-1α | − | − | − | − | − | − | − | − | − |
IL-1β | − | − | − | − | − | − | − | − | − |
IL-1ra | − | − | − | − | − | − | − | − | − |
IL-2 | − | − | − | − | − | − | − | − | − |
IL-4 | − | − | − | − | − | − | − | − | − |
IL-5 | − | − | − | − | − | − | − | − | − |
IL-6 | − | − | − | − | − | − | − | − | − |
IL-8 | − | − | − | − | − | − | − | − | − |
IL-10 | − | − | − | − | − | − | − | − | − |
IL-12 | − | − | − | − | − | − | − | − | − |
IL-13 | − | − | − | − | − | − | − | − | − |
IL-16 | − | − | − | − | − | − | − | − | − |
IL-17A | − | − | − | − | − | − | − | − | − |
IL-17E | − | − | − | − | − | − | − | − | − |
IL-18 | − | − | − | − | − | − | − | − | − |
IL-21 | − | − | − | − | − | − | − | − | − |
IL-27 | − | − | − | − | − | − | − | − | − |
IL-32α | − | − | − | − | − | − | − | − | − |
CXCL10 | − | − | − | − | − | − | − | − | − |
CXCL11 | − | − | − | − | − | − | − | − | − |
CCL2 | − | − | − | − | − | − | + | + | − |
MIF | + | + | + | + | + | + | + | + | + |
MIP-1α | + | + | + | + | − | +/− | − | − | − |
CCL5 | + | + | + | + | − | − | − | − | − |
CXCL12 | + | − | − | − | + | − | − | − | − |
Serpin E1 | − | − | − | − | − | − | − | − | − |
TNF-α | − | − | − | − | − | − | − | − | − |
TREM-1 | − | − | − | − | − | − | − | − | − |
We next examined the functional involvement of CCL5, MIP-1α and MIF in MDSC induction. In co-culture of PBMCs and KMS-12-BM or KMS-28-PE cells for 5 days, addition of anti-CCL5 neutralising Ab partially, but significantly, blocked MDSC induction, whereas addition of anti-MIP-1α Ab had no significant influence (Fig 2D; Fig S4). Moreover, supplementation with 1800 pg/ml of human recombinant CCL5 enabled MDSC-non-inducible RPMI8226, AMO1 and LP-1 cells to significantly increase MDSC fraction, whereas further addition of up to 600 pg/ml of MIP-1α did not increase MDSCs (Fig 2E). Although MIF was commonly secreted by all HMCLs examined, addition of a MIF inhibitor 4-iodo-6-phenylpyrimidine (4-IPP) significantly reduced MDSC induction, and the combination of anti-CCL5 Ab and 4-IPP almost completely abolished MDSC induction from PBMCs by co-culture with KMS-12-BM or KMS-28-PE cells (Fig 2F; Fig S4). Collectively, concomitant secretion of CCL5 and MIF, but not MIP-1α, from HMCLs may be a prerequisite for induction of MDSCs in a myeloma microenvironment.
In vitro pharmacological intervention in MDSC induction
LEN (10 µmol/l), POM (10 µmol/l), ATRA (5 µmol/l), MVC (10 µmol/l) or celecoxib (40 µmol/l) showed no anti-proliferative effects on the nine HMCLs (data not shown); however, clinically achievable 2·5 µmol/l LEN and 0·25 µmol/l POM significantly repressed MDSC induction from PBMCs, with POM being more potent than LEN. While the efficacy of LEN for MDSC inhibition seemed to be saturated at 2·5 µmol/l, POM up to 5 µmol/l showed a dose-dependent MDSC inhibitory effect (Fig 3A,B). Furthermore, we found that the pretreatment of MDSC-inducible HMCLs with LEN or POM for 48 h caused the prevention of MDSC induction from PBMCs by the co-culture (Fig S5). These collectively indicated that both LEN and POM directly acted on HMCLs to inhibit their MDSC-inducing properties. In addition, ATRA and MVC, but not celecoxib, inhibited MDSC induction (Fig 3B; Fig S6). While treatment with 1–5 nmol/l BTZ for 5 days seemed to reduce MDSC induction in a dose-dependent manner, this was actually accompanied by cell death induction in whole PBMCs. Due to this, the effect of BTZ on MDSC induction could not be exactly evaluated with our assay system which requires 5 days co-culture for MDSC induction (Fig 3C).
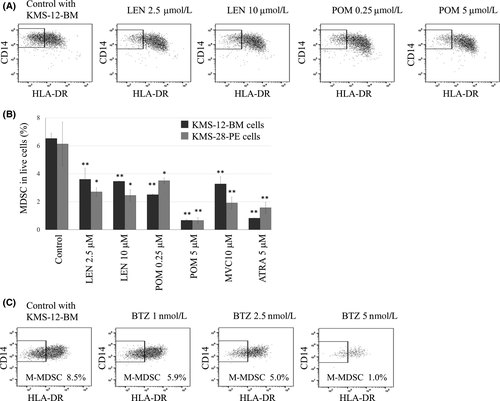
Mechanisms underlying the blockade of MDSC induction by IMiD compounds
We next explored mechanisms of blockade of MDSC induction by LEN and POM. LEN and POM at clinically achievable concentrations (i.e. as low as 0·1 µmol/l) significantly suppressed transcriptional expression and production of CCL5 and MIF of KMS-12-BM and KMS-28-PE cells (Fig 4A,B; Fig S7). As the critical target of IMiDs, we further investigated the role of CRBN in blockade of MDSC induction by LEN and POM. For this, we took advantage of PROTAC compounds, which selectively degrade CRBN without cytotoxicity. In a preliminary comparison of two PROTAC compounds, 15a and CRBN-6-5-5-VHL, chemical knockdown of CRBN was most effectively accomplished by 6-h treatment with 1 μmol/l CRBN-6-5-5-VHL in KMS-12-BM and KMS-28-PE cells (Fig S8). In addition, pretreatment with 1 μmol/l CRBN-6-5-5-VHL blocked degradation of IKZF1 and IKFZ3 by LEN or POM in myeloma cells. Using this system, we found that pretreatment of MDSC-inducible KMS-12-BM and KMS-28-PE cells with 1 μmol/l CRBN-6-5-5-VHL for 6 h mostly blocked downregulation of CCL5 transcription, but only partly (and not significantly) affected downregulation of MIF, by LEN and POM (Fig 4C,D). Regarding PBMCs, the surface expression level of CCR5, a receptor for CCL5, was significantly reduced by LEN and POM (Fig 5A,B). In addition, LEN and POM significantly increased the level of interferon regulatory factor 8 (IRF8) mRNA, a negative regulator of differentiation towards MDSCs (Fig 5C). Together, LEN and POM prevent MDSC induction through actions on both myeloma cells and normal PBMCs.
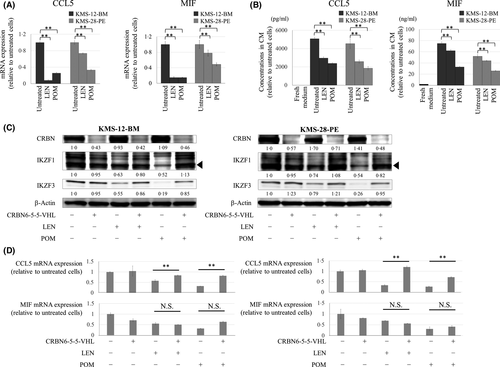
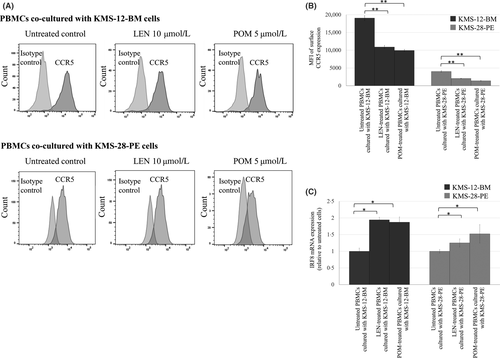
Serum CCL5 and MIF in relapsed/refractory MM (RRMM) treated by the combination therapy of carfilzomib, LEN and dexamethasone (KRD)
Finally, we investigated the impacts of KRD23 on serum levels of CCL5 and MIF in 10 patients with RRMM. All patients received KRD as salvage therapy for progressive disease, and achieved better than partial response within three cycles. Serum samples were obtained before treatment and after the third cycle of KRD. The mean (± SD) serum levels of CCL5 and MIF in five HDs were 53·7 (13·6) and 23·7 (5·9) ng/ml respectively. At baseline, neither CCL5 nor MIF was higher in serum from RRMM than from HDs. The significant reduction after KRD was observed in CCL5, but was not in MIF (Fig 6).
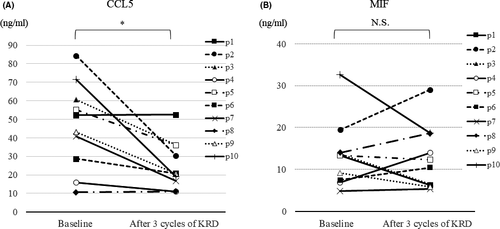
Discussion
Several studies have shown an association with disease progression and an increase of MDSCs in MM.11-13 Favaloro et al.11 showed an increase of PMN-MDSCs in PB of patients with MM along with disease progression, whereas Lee et al.13 found an association between an increase of M-MDSCs and a poor clinical effect of LEN. This discrepancy might be due to differences in patient backgrounds and treatment, and in methods of analysis of MDSCs. We found that M-MDSCs could be induced from normal PBMCs when co-cultured with specific HMCLs. However, our present result does not disprove the importance of an increase of PMN-MDSCs in MM shown in other studies, because changes in patient background and therapy might alter in vivo haematopoietic differentiation, including MDSCs.
Previous studies have shown that growth factors related to myelopoiesis, such as granulocyte macrophage-colony stimulating factor (CSF), granulocyte-CSF, and pro-inflammatory cytokines, including interleukin 6 (IL-6), IL-17, prostaglandin E2, tumour necrosis factor α (TNF-α), are involved in MDSC induction.1 However, none of these were involved in MDSC induction in our present assay. Instead, CCL5 and MIF secreted from HMCLs appeared to play roles in MDSC induction in MM. CCL5, a member of the C-C motif chemokine family, has roles in chemotactic cell migration of T cells, neutrophils, monocytes, eosinophils and basophils towards inflammatory sites, and has been implicated in promotion of MDSC induction and migration to tumour sites in several cancers.16, 24-26 Myeloma cells from advanced stage MM patients and HMCLs also express high levels of CCL5.
Moreover, CCL5 potently accelerates cancer cell growth, induces angiogenesis, and promotes tumour migration through interaction with its ligand CCR5.24 In the present study, MDSC induction was markedly reduced by anti-CCL5 neutralising Ab and by a CCR5 antagonist MVC,24 indicating that the CCL5-CCR5 axis is critical in MDSC induction by myeloma cells. MIF is a pro-inflammatory cytokine secreted by activated T lymphocytes, macrophages, and endothelial and epithelial cells, and is involved in macrophage migration, inflammation and immune response. Overexpression of MIF occurs in many solid cancers, in association with metastasis and MDSC production.28-30 MIF is also elevated in serum from patients with MM, and MIF overexpression associates with a poor prognosis and drug resistance.31 Among soluble factors involved in MDSC generation in various cancers, some are common regardless of the cancer type, while others are disease-type specific.1 The present study is the first to show the co-operative roles of CCL5 and MIF secreted by HMCLs are at least partly responsible for induction of MDSCs from PBMCs. However, the number of M-MDSCs induced from PBMCs by the supplementation of exogenous CCL5 into the co-culture with non-MDSC-inducible HMCLs was smaller than the number of M-MDSCs induced by the co-culture with MDSC-inducible HMCLs. These suggested the involvement of unknown factor(s), such as extracellular microRNA, in M-MDSC induction by the co-culture with HMCLs.
Development of a pharmacological strategy that targets MDSCs is an appealing research area in immuno-oncology,25 and several drugs approved for cancer or non-cancerous diseases have inhibitory effects on MDSCs.3, 16, 18, 19 Indeed, our present study recapitulated a previous finding of the in vitro anti-MDSC effect of ATRA, which might be mediated by glutathione synthase and the glutathione axis.2, 18 In contrast to MVC and ATRA, the mechanisms underlying the anti-MDSC effects of IMiD compounds has been unclear.13, 32 In the present study, LEN and POM showed distinct inhibition of MDSC induction without reducing normal PBMCs. Therefore, we conclude that LEN and POM have a unique molecular effect that prevents MDSC induction. IMiD compounds exert various immunomodulatory functions on immune cells, including cytotoxic T cells, Tregs, NK cells, and dendritic cells.32-38 Our present results showed that LEN and POM inhibit production of CCL5 and MIF from myeloma cells, with the former largely dependent on CRBN and the latter occurring mostly independent of CRBN. This indicates that CRBN-independent pathways have a role in the effects of LEN and POM in MM cells. While the nuclear factor kappa B (NF-κB) signalling pathway has been shown to promote CCL5 expression,24 it has been suggested that LEN blocks the NF-κB pathway in a CRBN-dependent manner.39 These imply the involvement of a CRBN-NF-κB-CCL5 cascade in CCL5 downregulation by IMiDs in HMCLs, whereas the molecular mechanism underlying the effect of IMiDs on MIF expression remains to be verified. Interestingly, our pilot investigation with 10 patients with RRMM suggested the possible involvement of serum CCL5 reduction, but not MIF, in IMiD-containing therapy. However, because the role of M-MDSC in LEN-containing treatment remains controversial,17 larger clinical investigation is expected to explore the significance of serum CCL5 level and M-MDSC as biomarkers for therapeutic response in MM, especially in the setting of various types of IMiD-containing immunochemotherapy.
We also found that LEN and POM acted on PBMCs and reduced CCR5 expression and induced IRF8 expression. IRF8 is an essential transcription factor for development of monocytic lineage cells,40 and recently was shown to inhibit MDSC differentiation.41 As the loss of CRBN expression in myeloma cells leads to resistance to LEN and an increase of MDSCs is related to LEN resistance,13, 42 there is also a need to investigate the clinical impact of CRBN expression in normal PBMCs in treatment with IMiD compounds of MM. In addition, discovery of a CRBN-independent strategy for eradicating MDSCs is required. Finally, it will be interesting to apply our present results on the effects of IMiD compounds on PBMCs to immunotherapy for other types of cancers.43
In conclusion, we explored the molecular mechanisms underlying MDSC induction by surrounding myeloma cells. IMiDs were identified as potent inhibitors of MDSC induction via overlapping mechanisms acting on myeloma cells and PBMCs (Fig 7). Our present findings may contribute to development of an immune profiling and monitoring method in IMiD compounds treatment in a clinical setting, as well to new therapeutic strategies in MM.
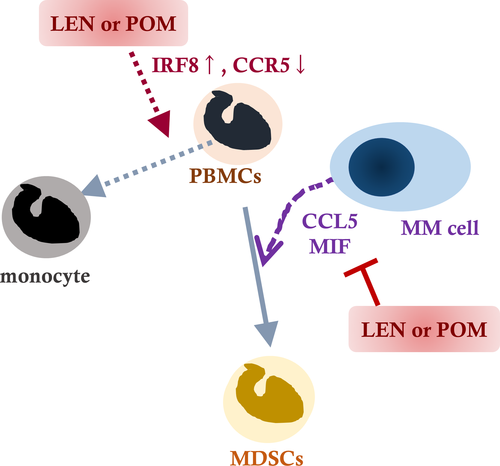
Acknowledgement
The authors thank Ms Sakamoto-Inada for her scientific support. The study was supported in part by National Cancer Center Research and Development Funds (26-A-4, 29-A-3), a Grant-in-Aid for Clinical Cancer Research (H26-kakushin-teki-gan-ippan-074) from the Ministry of Health, Labour and Welfare of Japan, by AMED (JP16ck0106077h003, JP17ck0106348h0001, JP18ck0106348h0002 and JP19ck0106348h0003), a research grant from Celgene Corporation (Junya Kuroda), a Grant-in-Aid for Young Scientists (B) (JSPS KAKENHI J182004103), and by a Japanese Society for Myeloma Research Award (Yuji Shimura).
Author contributions
Saeko Kuwahara-Ota, Yuji Shimura, Christian Steinebach, Reiko Isa, Junko Yamaguchi, Daichi Nishiyama, Yuto Fujibayashi, Tomoko Takimoto-Shimomura, Yoshimi Mizuno, Yayoi Matsumura-Kimoto, Taku Tsukamoto, Yoshiaki Chinen, Tsutomu Kobayashi, Shigeo Horiike, Masafumi Taniwaki, Michael Gütschow and Junya Kuroda performed experiments and analysed data. Saeko Kuwahara-Ota, Yuji Shimura and Junya Kuroda drafted the manuscript. Yuji Shimura and Junya Kuroda designed the study. Yuji Shimura, Michael Gütschow and Junya Kuroda designed experiments and supervised the research.
Conflict of interest
Junya Kuroda has received research funding from Celgene, Kyowa Kirin, Chugai Pharmaceutical, Ono Pharmaceutical, Sanofi, Eisai, Bristol-Myers Squibb, Sysmex, Astellas Pharma, Pfizer, Sumitomo Dainippon Pharma, Nippon Shinyaku, MSD, Fujimoto Pharmaceutical and Otsuka Pharmaceutical, has received honoraria from Janssen Pharmaceutical K.K, Celgene Corporation, Kyowa Kirin, Chugai Pharmaceutical, Ono Pharmaceutical, Sanofi, Eisai, Bristol-Myers Squibb, Astellas Pharma, Pfizer, Nippon Shinyaku, Sumitomo Dainippon Pharma, Fujimoto Pharmaceutical, Abbvie and Otsuka Pharmaceutical, and is a consultant for Janssen Pharmaceutical K.K, Celgene, Bristol-Myers Squibb, Sanofi and Abbvie. Masafumi Taniwaki has received research funding from Kyowa Kirin, Chugai Pharmaceutical, Eisai, Bristol-Myers Squibb, and Astellas Pharma. Tsutomu Kobayashi has received research funding from MSD, has received honoraria from Chugai Pharmaceutical, Ono Pharmaceutical, Eisai, and Nippon Shinyaku. Taku Tsukamoto has received research funding from Nippon Shinyaku.